How Negative Emission Technologies Are Fighting Against Climate Change
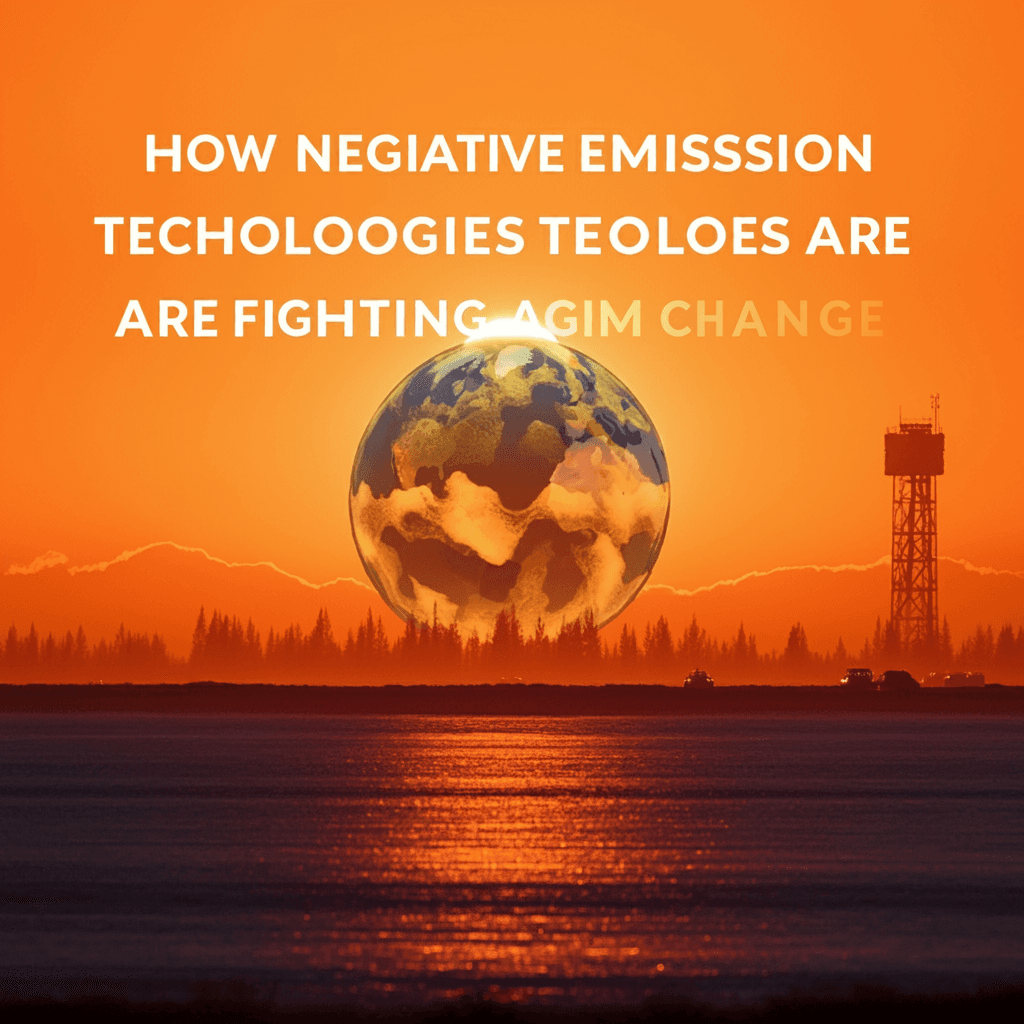
Addressing climate change demands not only reducing emissions but also removing existing carbon dioxide from the atmosphere. While some greenhouse gases, like methane, can be curbed, CO₂ levels have surged to record highs, calling for a strategy that goes beyond reductions alone. In sectors such as aviation and agriculture, achieving zero emissions is currently unfeasible, as these industries rely heavily on fossil fuels or land use that emits CO₂. Here, ""negative emissions" provide a pathway to balance out these unavoidable emissions, drawing greenhouse gases from the atmosphere to reach net-zero targets.
Negative emission technologies (NETs) are designed to tackle this balancing act. These include nature-based solutions like reforestation, which absorbs CO₂ naturally and advanced tech-driven approaches like Direct Air Capture (DAC), which captures carbon directly from the air. Imagine it as bailing out a boat taking on water faster than it leaks; just as a sinking boat requires both a fix and a way to expel the water, climate change mitigation needs both emission cuts and active carbon removal.
Projects like Iceland's Orca plant showcase the potential and limitations of DAC, capturing around 4,000 tons of CO₂ annually—a small fraction of the 10 gigatons per year scientists estimate are needed by 2050. Nevertheless, they remain a vital component in the climate solution framework, playing a key role in efforts to protect the planet. In addition to DAC, other NETs include
- Bioenergy with Carbon Capture and Storage (BECCS)
- Enhanced Weathering
- Afforestation
- Ocean-based carbon removal methods.
Here’s how each of them are fighting against climate change:
- Direct Air Capture (DAC)
Direct Air Capture (DAC) is an innovative technology designed to extract carbon dioxide (CO₂) directly from the atmosphere, regardless of its source. Unlike traditional carbon capture methods that typically work at the point of emissions, DAC captures CO₂ from ambient air, making it a versatile solution for addressing dispersed emissions.
The DAC process typically uses large fans to move air through systems that contain chemical sorbents—such as liquid solvents or solid sorbents. These sorbents selectively capture CO₂ molecules from the air. Once the CO₂ binds to these chemicals, it is separated and concentrated, then transported for either permanent storage or usage in other industrial processes. One common method of sequestration is storing the captured CO₂ underground in geological formations, ensuring long-term removal from the atmosphere. Alternatively, the concentrated CO₂ can be repurposed for synthetic fuels or other carbon-based products, contributing to a circular carbon economy.
While DAC holds promise, the technology is still developing due to high energy and operational costs. However, as renewable energy sources become more affordable, the feasibility of DAC systems will likely increase, potentially scaling up to be a significant contributor to net-zero goals.
- Bioenergy with Carbon Capture and Storage (BECCS)
Bioenergy with Carbon Capture and Storage (BECCS) is a two-step approach to negative emissions that involves generating energy from biomass and then capturing and storing the resultant CO₂. Biomass—organic material such as wood, crop residues, and other plant materials—absorbs CO₂ from the atmosphere as it grows. When this biomass is burned for energy, the process releases CO₂. However, by incorporating carbon capture and storage, BECCS captures this CO₂ before it is emitted back into the atmosphere.
The captured CO₂ can be stored in secure geological formations or deep saline aquifers, resulting in a net reduction of atmospheric CO₂. Since the plants initially absorbed CO₂ during their growth phase, the process effectively removes carbon from the air. Moreover, BECCS is versatile and can be applied to various bioenergy systems, including power plants, biofuel production, and other biomass combustion processes.
Although BECCS holds great potential, it also faces challenges, such as the need for significant land resources to grow biomass, competition with food production, and possible ecological impacts. Sustainable management of land and careful selection of biomass sources are crucial to ensure that BECCS can contribute effectively to negative emissions without unintended consequences.
- Enhanced Weathering
Enhanced weathering is a natural process that accelerates the removal of carbon dioxide from the atmosphere. In nature, certain types of minerals, such as basalt or olivine, gradually react with atmospheric CO₂ in a process called mineral carbonation. Enhanced weathering involves spreading finely ground silicate minerals on large surfaces, like agricultural lands, where they can react with CO₂ at an accelerated rate.
When these minerals come into contact with water, they chemically react with CO₂ to form stable carbonate minerals that can permanently lock away carbon for hundreds to thousands of years. Enhanced weathering thus provides a low-risk solution for long-term CO₂ storage, as the resulting carbonates are stable and remain sequestered without requiring additional infrastructure.
One potential benefit of enhanced weathering is that the process can also help to improve soil quality and counteract soil acidification, benefiting agricultural productivity. However, it requires mining, grinding, and distributing large quantities of minerals, which has associated costs, energy requirements, and environmental considerations. Research into optimising mineral types and distribution methods is ongoing to make enhanced weathering a viable NET on a large scale.
- Afforestation
Afforestation, or planting trees in areas that previously had no forest cover, is one of the most well-known and natural methods of removing CO₂ from the atmosphere. Trees and other plants absorb CO₂ during photosynthesis, using the carbon to grow while releasing oxygen back into the atmosphere. Establishing forests on land previously unused or cleared for other purposes, and afforestation helps to expand carbon sinks and improve biodiversity.
As the trees grow, they sequester carbon in their trunks, branches, roots, and surrounding soil, providing a long-term reservoir for atmospheric CO₂. Afforestation projects can be strategically implemented in degraded lands or marginal areas that are not suitable for agriculture, helping to prevent competition with food production.
Afforestation does face challenges: land availability, water needs, and the risk of reversal due to forest fires, disease, or changes in land use. Additionally, careful selection of native tree species and ecological management practices are essential to maximise benefits for biodiversity and climate resilience.
- Ocean-Based Carbon Removal Methods
The ocean, which naturally absorbs large amounts of CO₂, can be utilised in various ways to enhance carbon removal through ocean-based methods. Some promising techniques include ocean fertilisation, alkalinity enhancement, and the cultivation of marine biomass.
- Ocean Fertilization: This approach involves adding nutrients, such as iron, to specific areas of the ocean to stimulate phytoplankton growth. Phytoplankton, like terrestrial plants, photosynthesise, taking in CO₂ from the surrounding water. When they die, a portion of this CO₂ may sink to the ocean floor, sequestering carbon for long periods. However, ocean fertilisation requires careful monitoring, as it can lead to ecosystem imbalances and potentially harmful algal blooms.
- Alkalinity Enhancement: Increasing the alkalinity of seawater through the addition of minerals like limestone enhances the ocean’s capacity to absorb CO₂.. The dissolved CO₂ is converted to bicarbonate, a stable form that does not re-release into the atmosphere. Alkalinity enhancement could help neutralise ocean acidification, creating a dual benefit of carbon sequestration and ocean health improvement.
- Marine Biomass Cultivation Growing and harvesting seaweed or algae on a large scale could capture significant amounts of CO₂. Seaweed absorbs CO₂ as it grows, and certain types can be harvested and stored in deep ocean locations, effectively sequestering carbon. Seaweed farms could also provide additional environmental benefits, like supporting marine biodiversity and reducing local ocean acidification.
Challenges With Negative Emission Technologies (NETs) In Africa
Negative Emission Technologies (NETs) are often viewed as promising tools for addressing global climate change, but from an African perspective, they present unique and complex challenges. In many parts of Africa, where communities rely heavily on land for food production, livelihoods, and cultural practices, large-scale deployment of NETs such as Bioenergy with Carbon Capture and Storage (BECCS) and afforestation projects can come at a high social and economic cost.
For example, BECCS requires extensive land and water resources to cultivate the biomass necessary for carbon removal. Implementing this technology on a large scale could intensify competition for arable land, which is already a precious resource across much of Africa. Countries where agriculture plays a central role in both the economy and food security could face serious trade-offs if vast tracts of land are reallocated for biomass cultivation. This reallocation may compromise local food systems, displace rural communities and their indigenes, and disrupt traditional land-use practices, impacting food sovereignty and community stability. In this context, NETs risk being perceived as benefiting the global climate agenda at the expense of local well-being, raising important ethical and equity concerns.
The economic costs associated with NETs present another major challenge. Technologies like Direct Air Capture (DAC) and enhanced weathering demand advanced infrastructure and significant energy inputs, often derived from renewables to ensure the process is carbon-neutral. However, many African nations are still working to expand renewable energy access for households and industries, and diverting these resources to support NETs could hinder local energy security efforts. Given the high upfront costs and maintenance demands, NETs may be financially out of reach for many African countries without substantial international support. This economic disparity raises questions about whether deploying NETs in Africa would create disproportionate financial burdens, potentially exacerbating existing inequalities between nations in the Global South and those in the Global North.
From a policy perspective, NETs require robust frameworks to monitor and manage their deployment. However, many African countries currently lack the regulatory systems needed to oversee the implementation of these technologies effectively. Without clear governance structures, there is a risk that NETs could be deployed in ways that overlook or undervalue local communities' rights, resources, and livelihoods. Additionally, the global reliance on NETs may create a moral hazard, where developed countries use African lands to offset emissions rather than focusing on their own reductions, shifting the burden onto nations that are already disproportionately affected by climate impacts.
In light of these challenges, it’s crucial for NETs to be viewed as complementary, rather than primary, solutions in Africa's climate strategy. They should support—not replace—emission reduction efforts, and they must be integrated in ways that prioritise local resilience, equity, and sustainable development. For Africa, a balanced approach that combines emission reductions with carefully chosen, community-inclusive NETs could help advance climate goals while protecting the social, environmental, and economic foundations essential for future growth and stability.